What is Thermodynamics?
Thermodynamic is the branch of science which deals with concepts of heat and temperature and the inter conversion of heat and other forms of energy.
The word “thermodynamic” actually meant for “heat in motion. “Therm” means “heat” and “dynamics” means “in motion”.
Thermodynamic System
In thermodynamic point of view, an universe is consisting of two components viz. –
- System.
- Surroundings.
System
A part of universe which is under the observation and consideration of investigation or study is called a thermodynamic system.
- A system is consisting of two important components of prime consideration – (1) Mass and (2) Energy.
- A system is an assembly of a very large number of particles whose status is expressed in certain values – (1) Pressure (2) Volume and (3) Temperature.
Surrounding
Remaining part of universe which is left other than the thermodynamic system is called its surroundings.
Therefore, an universe is the total sum of a system and its surroundings.
Therefore, \quad \text {Universe} = \text {System} + \text {Surrounding}
Boundary
Anything that separates a thermodynamic system from its surroundings is called a boundary.
Depending upon the flexibility, a boundary is classified into two types –
- Real boundary – It is a rigid boundary which can’t move. The size of a system in real boundary is fixed. (e.g. gas kept in a bottle)
- Flexible boundary – The size of a flexible boundary can vary with time. It is of two types.
- Real flexible boundary – It is the flexible boundary which can expand in shape and size. (e.g. heating of gas in a balloon, diaphragm, cycle tubes)
- Rigid movable boundary – It is the rigid boundary which can move to change the size. (e.g. gas contained in a piston and cylinder arrangement).
Depending upon behavior with transfer of heat energy, a boundary is of two types.
- Diathermic boundary.
- Adiabatic boundary.
(1) Diathermic boundary
It is a heat conducting boundary which permits heat exchange between system and surroundings.
Example – A gas flowing through tubes of heat exchanger or condenser.
(2) Adiabatic boundary
It is a heat non-conducting boundary and no heat exchange occurs between system and surroundings.
Example – Liquid kept in a thermos flask.
Types of Thermodynamic Systems
Depending upon the interaction of system particles with surroundings, a thermodynamic system is divided into three categories –
(1) Open system
An open system has no distinct boundary surface between system and surroundings.
In an open system –
- Mass exchange occurs between system and surrounding.
- Energy exchange also occurs between system and surrounding.
Example –
- Water boiling in open vessel.
- A hot body kept in open atmosphere and get cooling down.
(2) Closed system
A closed system has a distinct but conducting boundary surface.
In a closed system –
- Mass exchange is restricted between system and surrounding.
- Energy exchange occurs between system and surrounding.
Example –
- Water boiling in closed vessel having a lid.
- Cooking of food in pressure cooker.
(3) Isolated system
An isolated system has a distinct non-conducting boundary surface.
In an isolated system –
- Energy exchange is restricted between system and surrounding.
- Mass exchange is also restricted between system and surrounding.
Example –
- Hot tea or coffee kept in a thermos flask.
- Expansion of a gas contained in a piston cylinder arrangement whose all surfaces are non conducting.
Thermodynamic Properties
Properties which help us in the study of behavior of a thermodynamic system are called thermodynamic properties.
Thermodynamic proprieties are of two types –
- Extensive or extrinsic property.
- Intensive or intrinsic property.
Extensive Property
- These are the properties which depend upon mass of the system.
- These depend upon matter content and size of a system.
- As the matter contained or size of the system change these properties also get change.
Example – Mass, volume, number of moles, heat capacity etc. are extensive properties.
Intensive Property
- These are the properties which are independent of mass.
- It does not depend upon matter content or size of the system.
- These properties doesn’t change with change in size of the system.
Example – Density, refractive index, specific heat, concentration, pressure, temperature, viscosity etc. are intensive properties.
TO BE NOTED –
It may be possible that, ratio of two extrinsic properties give an intrinsic property. For example – Number of moles and volume, both are extrinsic properties. But their ratio called “Molarity” is an intrinsic property.
Thus, \quad \text {Molarity ( Intrinsic )} = \left [ \frac {\text {Number of moles of solute ( Extrinsic )}}{\text {Volume of solution ( Extrinsic )}} \right ]
Other similar examples are Normality, mole fraction, dielectric constant, electromotive force etc.
Thermodynamic Variables
A thermodynamic process may involve different types of variables. The followings are the most common thermodynamic variables –
- Temperature of the system and surroundings.
- Pressure of the system and surroundings.
- Volume of the system.
- Heat content, enthalpy and entropy of the system.
- Work done by the system.
- Energy and Gibb’s function of the system.
- Mass of the system.
- Concentration of the system i.e. Mole fraction, Normality, Molarity, Molality etc.
Thermodynamic variables are divided into two categories – (1) State function and (2) Path function.
State Function
State functions or state variables are the thermodynamic properties which only depend upon the initial and final states of a system.
- State functions doesn’t depend upon the actual path followed by the system.
- In a cyclic process, the total change in state functions is zero.
Examples –
Pressure ( P ) , volume ( V ) , temperature ( T ) , mole number ( n ) , enthalpy ( H ) , internal energy ( U ) , Gibbs free energy ( G ) etc. are state functions.
Path Function
Thermodynamic properties which depends upon the actual path followed by the system are called path functions or path variables.
- Path functions depend upon the actual path followed by the system.
- In a cyclic process the total change in path functions is not zero.
ILLUSTRATION
Consider about a cyclic process as shown in figure.
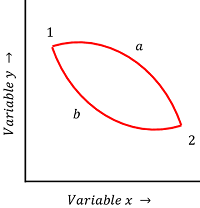
- A system is initially at state ( 1 ) and after going through the path ( a ) , it reaches to final state ( 2 ) .
- Let, through another process via another path ( b ) , the system reach the same final state ( 2 ) .
As pressure, volume and temperature of the system are state functions, we get the same values at point ( 2 ) via either of the two paths ( a ) and ( b ) .
Therefore, \quad ( P_{2 \text {via a}} = P_{2 \text {via b}} ) \quad ( V_{2 \text {via a}} = V_{2 \text {via b}} ) \quad ( T_{2 \text {via a}} = T_{2 \text {via b}} )
But, heat ( Q ) and work ( W ) are path functions. Hence, their values will be different for the process occurred via path ( a ) or via path ( b ) .
Therefore, \quad ( Q_{2 \text {via a}} \ne Q_{2 \text {via b}} ) \quad ( W_{2 \text {via a}} \ne W_{2 \text {via b}} )
Examples –
Heat ( Q ) and work ( W ) etc. are path functions.
Reversibility of a Process
Thermodynamic processes can be of two types –
- Reversible process.
- Irreversible process.
Reversible Process
A reversible process is defined as a process which can be reversed by varying in its conditions so that every change occurring in direct process is exactly reversed in the reversible process.
A reversible process has following features –
- At the end of the process both the system and surrounding return to their initial state.
- The process is a quasi-static process i.e. the direct process must be carried out in infinitesimally slowly so that the system remains in thermal equilibrium all the time and mechanical equilibrium at all positions throughout the process is maintained.
- The dissipating forces such as viscosity, friction, elasticity etc. are absent in a reversible process.
- These are ideal processes and difficult to achieve in real processes.
Reversibility is a basic concept of thermodynamics.
Thermodynamic mainly deals with efficiency or performance by which a heat engine converts heat energy into work energy.
By second law of thermodynamics, no heat engine can have efficiency of 100 \% . But it is possible to have maximum efficiency for a heat engine working between temperatures range ( T_1 ) and ( T_2 ) .
An ideal heat engine based on a reversible cyclic process can have the highest possible efficiency.
Example –
A Carnot engine is an Ideal reversible engine.
- A Carnot engine works on the principle of Carnot cycle.
- A Carnot engine has the maximum possible efficiency for a heat engine.
- All practical engines have efficiency lower than the Carnot efficiency.
Hence, reversibility is a basic and important concept for understanding thermodynamic cycles.
Irreversible Process
Any process which cannot be reversed in exact reversed direction by varying its conditions, is called an irreversible process.
- Most of the processes occur in nature are irreversible process.
- An irreversible process occurs very fast so that the system doesn’t get enough time to maintain thermal equilibrium and mechanical equilibrium at all positions throughout the process.
- The dissipating forces such as viscosity, friction, elasticity etc. are present in real processes that affect the performance of the process.
- These are real processes.